1. Consider other aspects of municipal solid-waste
The generation of municipal solid waste (MSW) is continuously increasing globally. The increase in urban solid waste generation is determined to be due to various factors such as economic growth, population expansion, industrial development, urbanization, migration from rural to urban areas, etc. Along with the increase in waste volume, the composition of urban solid waste has also become increasingly heterogeneous and complex due to the development of modern consumer-centric.
Along with the generation of MSW and the need for sustainable management, the global energy demand has also increased. Renewable energy sources for heat, electricity and various forms of biofuel production have become a top priority in national and worldwide energy policies. MSW highly assesses its use as a renewable energy source.
The term WtE (Waste to Energy technology) refers to the treatment of waste to recover energy from heat and electricity or other alternative fuels in gaseous, liquid, and solid states.
2. Waste-to-energy (WtE) pathways
There are three main WtE conversion pathways: thermal, biochemical, and physicochemical. However, there are different types of WtE process technologies, and each technology and process follows one of three transfer pathways.
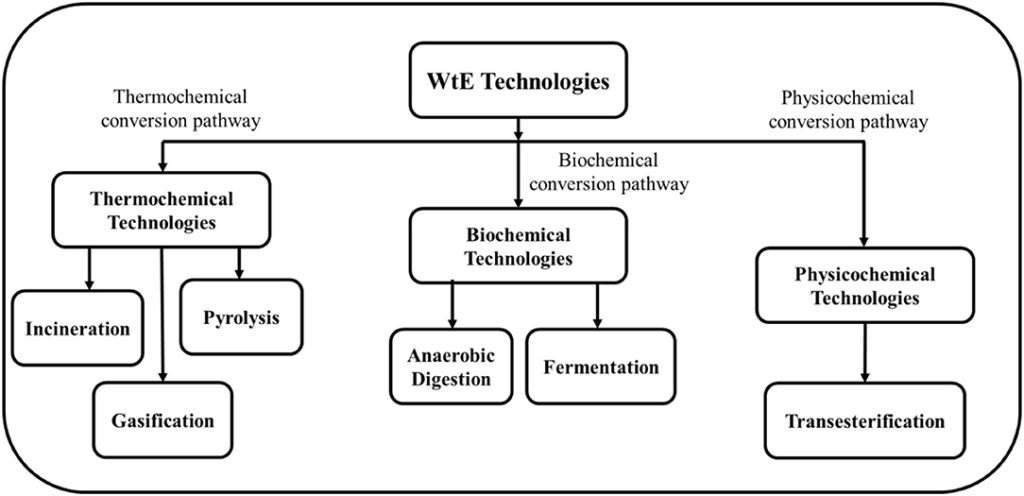
Figure 1. Classification of WtE technologies related to the conversion path
2.1. Thermochemical technology
The mechanism of the thermochemical conversion pathway is that thermal energy is needed to break down the molecular structure of municipal solid waste components and convert larger molecules into smaller molecules. The additional oxygen is then supplied in combination with hydrogen and carbon atoms released from large waste molecules that have decomposed and produce more energy than is provided to break down the structure. The characteristic of technologies that follow the path of thermochemical conversion is the use of very high temperatures to convert various small parts of municipal solid waste into heat, electricity, and other value-added products. These technologies include combustion, pyrolysis, and gasification. In particular, combustion is one of the most commonly used WtE technologies globally.
2.2. Biochemical technology
The biochemical conversion of biochemicals into energy involves biological agents or microorganisms like yeast, to convert the organic part of the waste into biofuel in gaseous form or liquid form. Anaerobic decomposition and fermentation are WtE technologies that follow the path of biochemical transformation. Inside:
- Anaerobic decomposition: with the help of microorganisms in the absence of free oxygen, the process of deterioration of the organic part of municipal solid waste occurs. Biogas generated during anaerobic decomposition can be used to replace natural gas during heat and energy. However, the efficiency of heat and electricity production from biogas is about 5.5-7.5kW/p, lower than that of natural gas. Liquid decomposers and fiber produced during anaerobic decomposition can be used as secondary raw materials in the fertilizer industry.
- The fermentation process also uses microbiology to decompose organic materials in an oxygen-free environment. It is a metabolic process carried out under conditions that are not disinfected using yeast or bacteria to convert sugars into alcohol. The ethanol application can be used to replace gasoline as a CO2 transport fuel that can be liquefied and sold as dry ice to the confectionery or processed food industries to generate additional revenue, etc.
2.3. Physicochemical technology
It is the process of converting the fat portions of food waste in the MSC stream into biodiesel. Ester metabolism is the most well-known WtE technology that follows the path of physicochemical conversion and conversion of food waste, especially used cooking oils (UCO) and fat levels. Ester metabolism is the most well-known WtE technology that follows the path of physicochemical conversion and conversion of food waste, especially used cooking oils (UCO) and animal fats into liquid fuels (biodiesel). Biodiesel can replace petrochemical diesel and use as vehicle fuel.
3. LCA Environmental Assessment Tool - Life Cycle Assessment
Environmental assessment tools are becoming more important due to the growing concern about including the environmental impacts of different products and production systems for sustainability in the industry. LCA is one of many tools that can be considered the broadest environmental assessment tool.

Figure 2. LCA tool model
It is a valuable tool for documenting environmental considerations over the life cycle of a product, service, commodity, or project that needs to be part of a decision-making process toward sustainability. LCA assesses important environmental aspects and their impact and extraction process on production use, and end-of-life stages, thus looking at the entire life cycle – from infancy (from when procuring raw materials through production use, and disposal).
LCA helps avoid the problem of moving through different stages of the production system by taking into account upstream and downstream processes and extending the focus beyond the physical boundaries. It also quantifies all relevant emissions, consumed and depleted resources, and covers a wide range of impacts related to every stage of the product’s life. Over the last decade, LCA has been widely used for the environmental assessment of WtE technologies both as a stand-alone technology and as part of an integrated urban solid waste management system. Example using product life cycle assessment (LCA) for environmental impact assessment of centralized biogas production system from municipal solid waste in buildings.
Reference: A Framework for the Selection of Suitable Waste to Energy Technologies for a Sustainable Municipal Solid Waste Management System – Ahsan Farooq, Piyanon Haputta, Thapat Silalertruksa and Shabbir H.Gheewata.
Author: Do Thi Hue